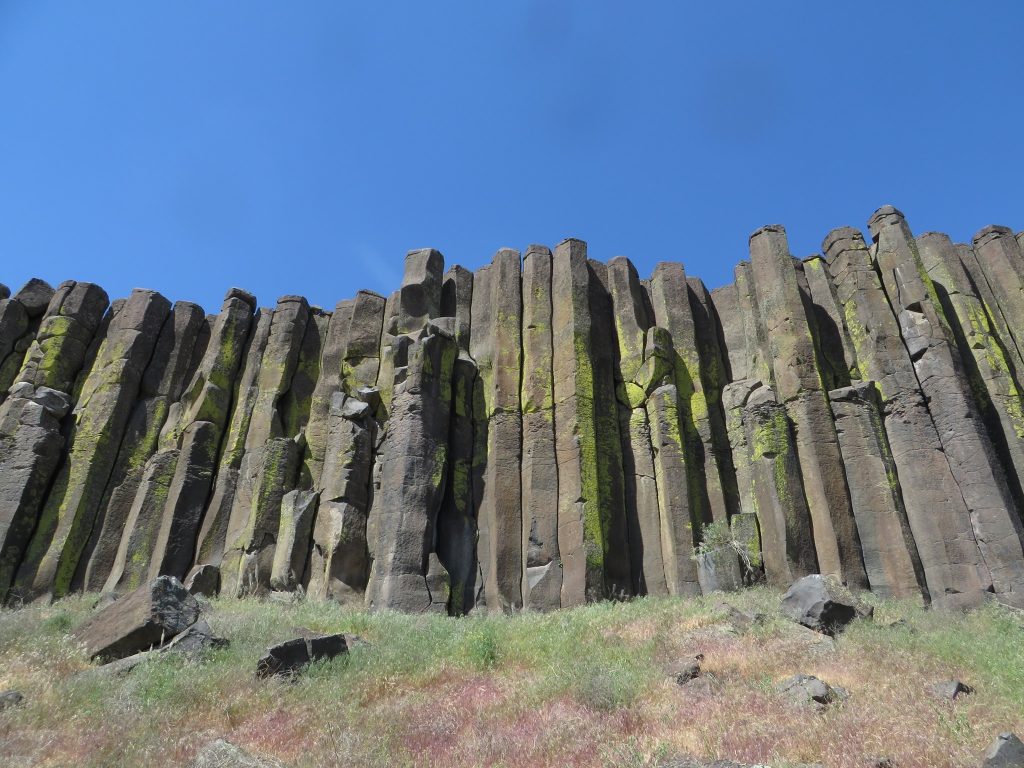
I could start with this: CaMgO6Si2. What does it tell you about basalt? Not much, if you don’t know how to read it. Some important things, if you do. But I am not a chemist, and this is not a textbook, so I will not delve into the proportions of pyroxenes, olivine, and silica. I won’t talk about the differences between basalt, gabbro, andesite, and rhyolite. I’ll keep it simple: Basalt is lava. It is a certain kind of lava. It is common on the face of the earth, and even more common on the floor of the sea. It possesses some interesting characteristics. And it can be beautiful.
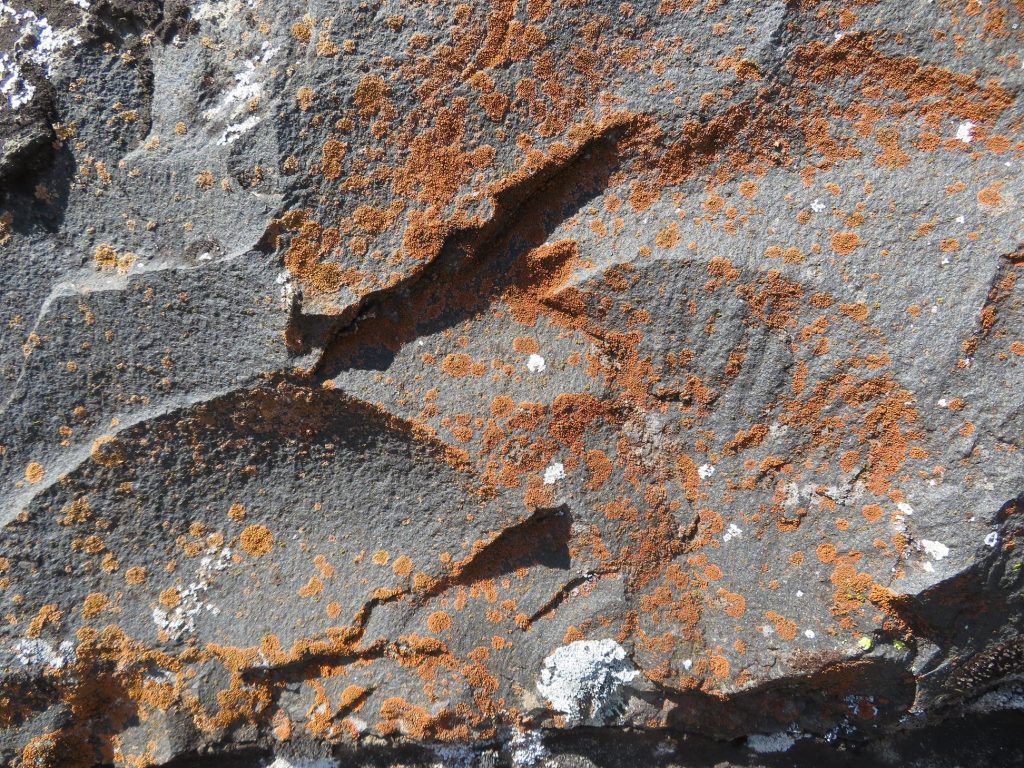
Basalt is the most common bedrock on the face of the earth, although most of it is on the ocean floor. On land, it accounts for about 10% of the earth’s surface. Most of it occurs in large provinces known as “flood basalts,” places where huge amounts of basaltic lava erupted, over an extended period, above a “hot spot.” A hot spot is a place where a plume of magma rises through the earth’s mantle, and finds its way to the surface. The word plume sounds sort of sort of playful and non-threatening. Delicate, even. Plume is the French word for feather. It is easy to imagine a feather of magma tickling the underbelly of the earth’s crust. The word conveys neither the volume of magma involved, nor the consequences of its entry into the surface world.
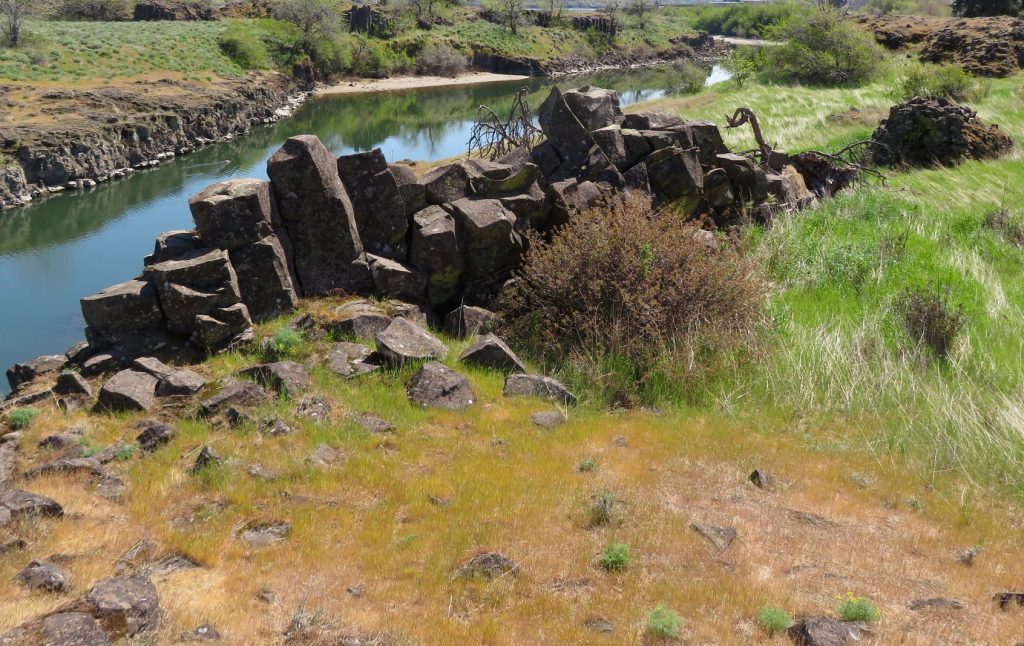
Hawaii is one such hot spot. The magma plume remains stationary, but the earth’s crust slides over it, creating the impression that the hot spot is moving. The Big Island is currently located right over the hot spot; in the past, it was Maui, and before that, Molokai, then Oahu, and so on. The string of islands stretching to the northwest leaves a record of how the tectonic plate has moved. Yellowstone also sits over a hot spot. A series of ancient calderas lined up between Yellowstone and northern Nevada shows us how the hot spot has moved—or, rather, how it has has not moved, but the earth’s skin has moved over it. The reason Yellowstone is sometimes referred to as a potential “supervolcano” is because the amount of magma that can be brought to the surface by a mantle plume is incredibly large—far larger than the amount of magma that lies beneath a subduction-zone volcano.
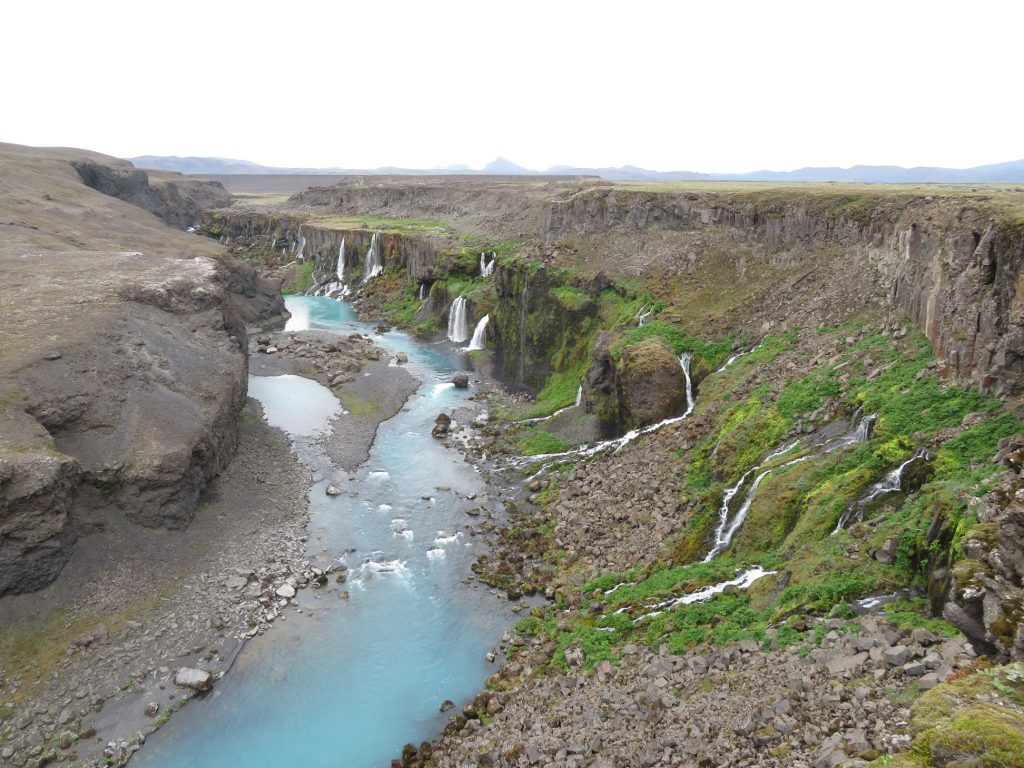
I suspect that in the imaginations of most people, the archetypical volcano is a glimmering cone, like Mount Fuji or Rainier, or Cotopaxi. Most of the planet’s famous volcanoes belong to mountain chains that parallel plate boundaries. Along a plate boundary, one tectonic plate dives beneath another, partially melting in the process. This fuels the volcanoes. But despite their beauty and fearsome eruptions, these subduction zone volcanoes are not the sources of most of the earth’s basalt. Other volcanoes rise right in the middle of a tectonic plate, far from a subduction zone. The explanation is a hot spot. The lava might break through via explosive eruptions, but it also might come through a series of cracks or rifts that bleed lava in huge amounts over thousands of years. These may not be as impressive as a towering mountain, but over time they are responsible for most of the lava on the earth.
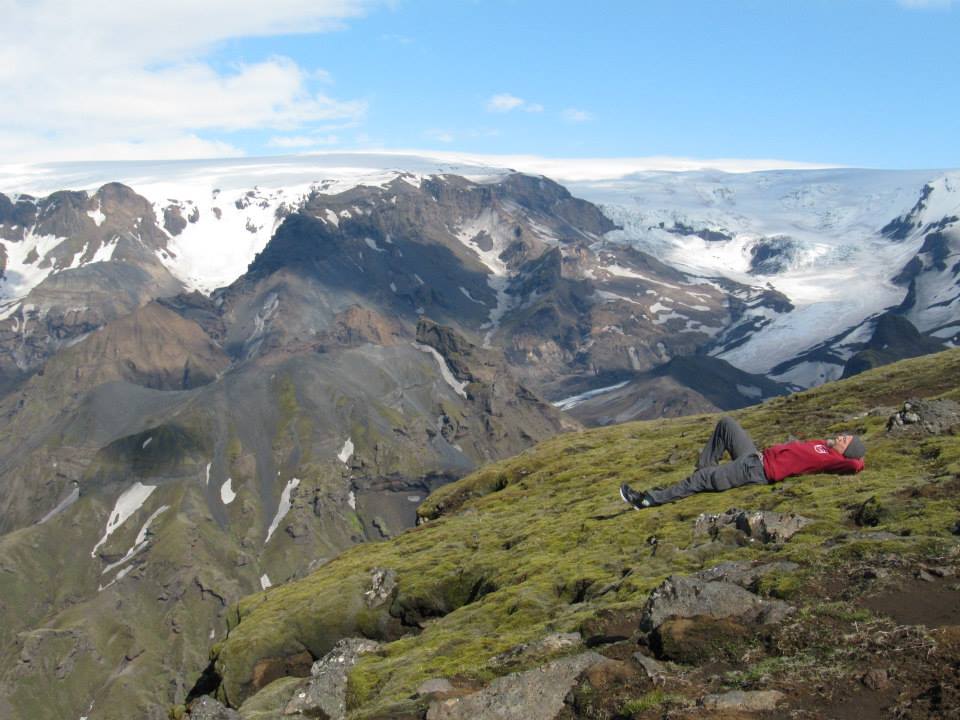
Iceland sits directly above another hot spot. In fact, the island is only one small part of an enormous area of flood basalts, most of it under water. This area—known as the North Atlantic Large Igneous Province—spans the Atlantic, from the coast of Greenland to the coast of Norway. This hot spot, however, is unique in one respect; instead of being in the middle of a tectonic plate, it occurs right along the boundary of two plates that are spreading apart. This means the volcanoes of Iceland are fueled by two sources: a hot spot and a separating seam. As a result, Iceland is particularly active. In the past 800 years, one third of the lava that has flowed over the earth’s surface has been on the island of Iceland.
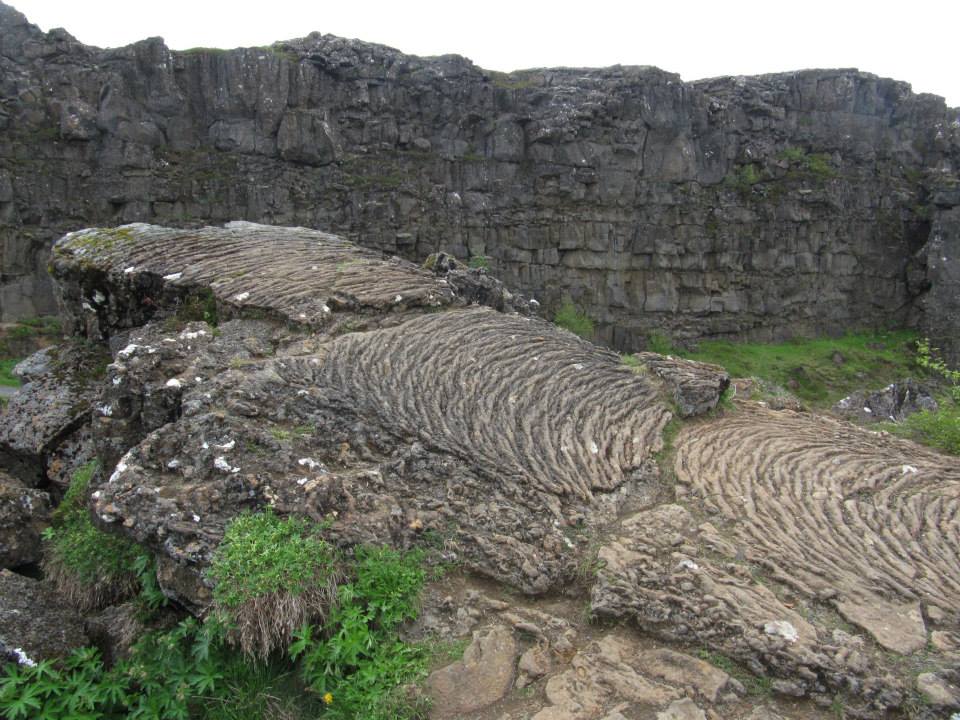
There are Large Igneous Provinces (LIPs) all over the world. Most of them are on the sea floor, but a few well-known LIPs are on land. The largest of these regions is an area in Russia called The Siberian Traps. This region is roughly the size of Alaska. Another well-known LIP covers about one-fourth of India and is known as The Deccan Traps. Why are these geological landforms called Traps? The word is derived from the Swedish word for step. Over thousands of years, the basalt was deposited in multiple layers. On the flank of a mountain or canyon, each of these layers has the appearance of a terrace, or a step.
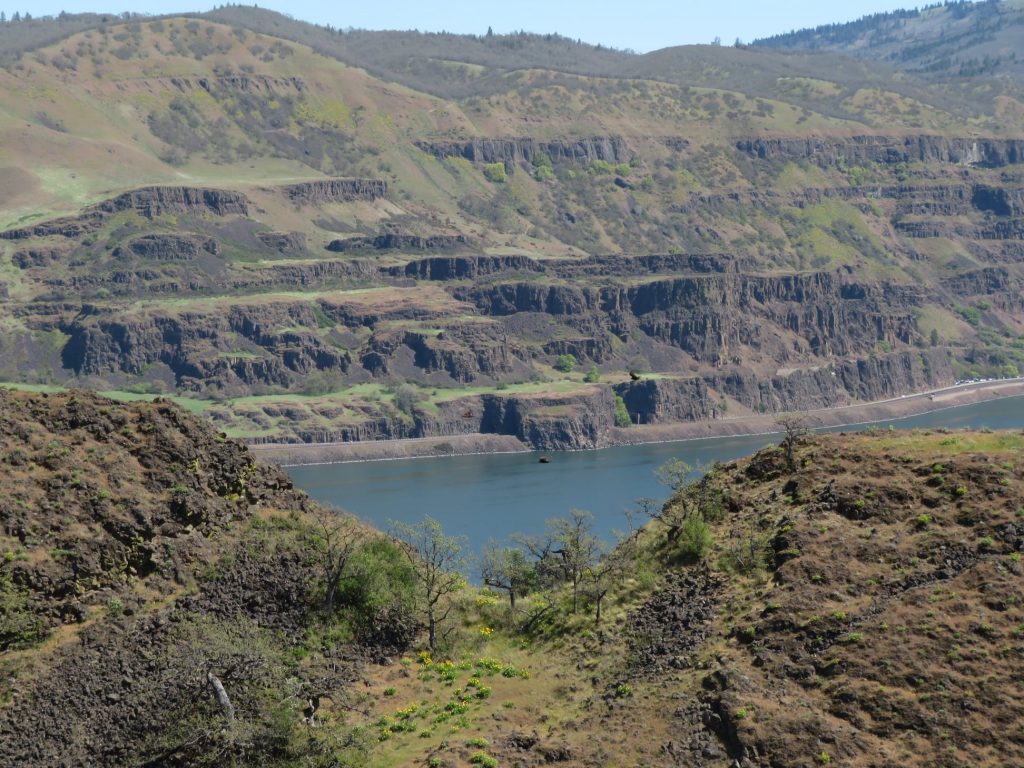
In the United States, we have our own homegrown LIP, the Columbia Basin flood basalts, which cover much of Washington, Oregon, and Idaho, and a small slice of Nevada as well. It is the largest expanse of basalt in North America. As massive as it is, however, it is small compared to the Siberian Traps. It is difficult to be precise about the volume of lava that came from the eruptions that created the Siberian Traps, but geologists have estimated that it could have covered all of Western Europe to a depth of one kilometer.
It could take an entire book to really talk about basalt. It merits such attention. Like water, it is both ubiquitous and fascinating. People take it for granted. (They may also take it for granite.) But I am not a geologist, and I’m only writing a blog entry. This is not a Large Igneous Lecture (LIL). It is a Small Igneous Blog Entry (SIBE). It is also an excuse to share some photography. So it is high time that I focused on the beauty of basalt in one of its common forms: the column.
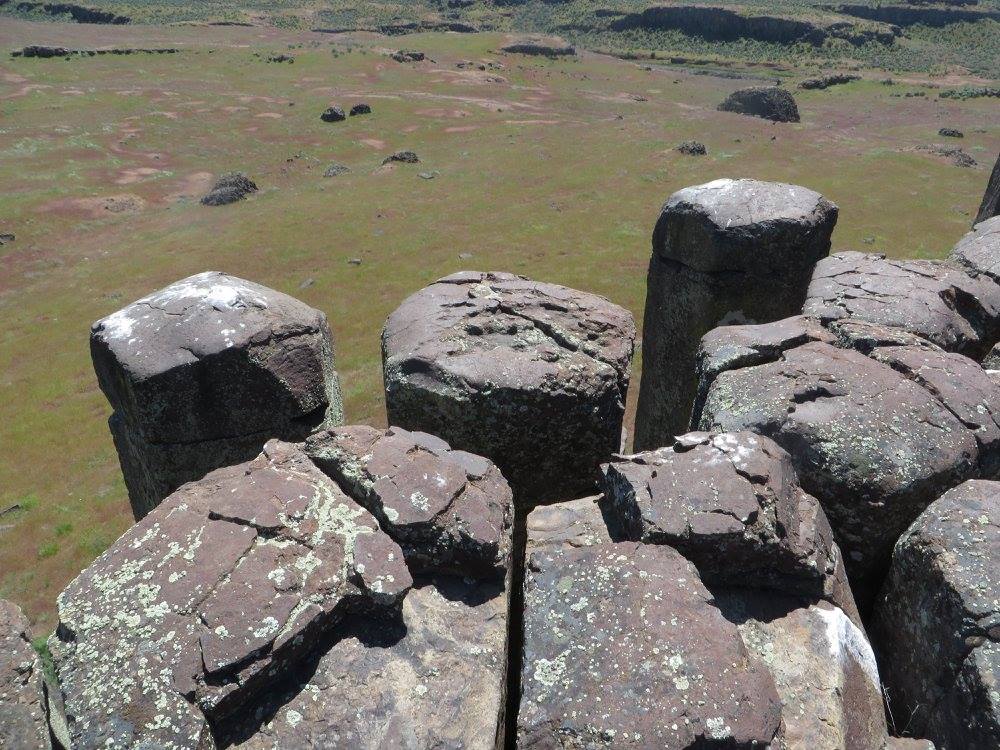
Based on the circumstances of its creation, basalt is diverse in texture, color, and form. Sometimes it solidifies into columns; other times it is amorphous, ropy, or frothy. Sometimes it is dense; other times it is full of holes from trapped air bubbles. In places where recurring lava flows occurred over thousands or millions of years, it is often stratified, like sedimentary rock, with each layer displaying distinct characteristics due to a different rate of cooling or different proportions of mineral ingredients.
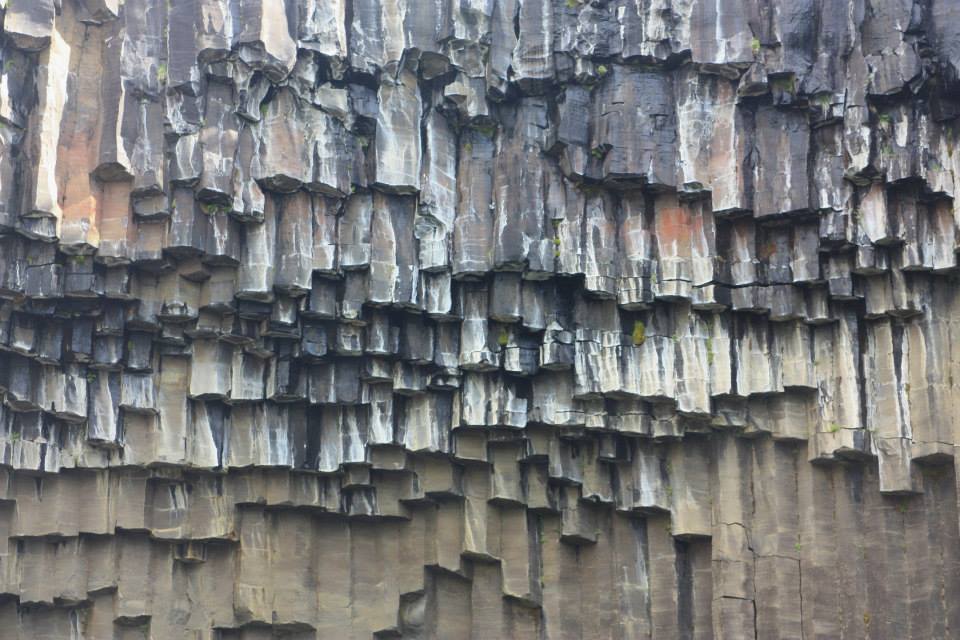
Columnar basalt is formed when a thick lava flow cools slowly. Lava that is in immediate contact with air or water will not form columns, but interior lava that is insulated has more time to cool. As it cools, the lava contracts. Cracks develop, just as they do in the mud of a drying lakebed when the perimeter of the lake does not shrink, but the mud within the perimeter does. The cracks do not occur in random fashion; as it turns out, nature prefers an angle of 120 degrees. And this creates hexagons. The thicker the flow, the longer it takes for lava to cool. The longer it takes to cool, the larger in diameter the columns will be. If the cooling of the lava was uniform throughout the flow, the hexagons would be perfect, but seldom is cooling uniform. With uneven cooling, the hexagons are distorted and irregular. Of course, some columns just feel a need to be different, and so pentagons occur from time to time. Nature is a dance of pattern and variation.
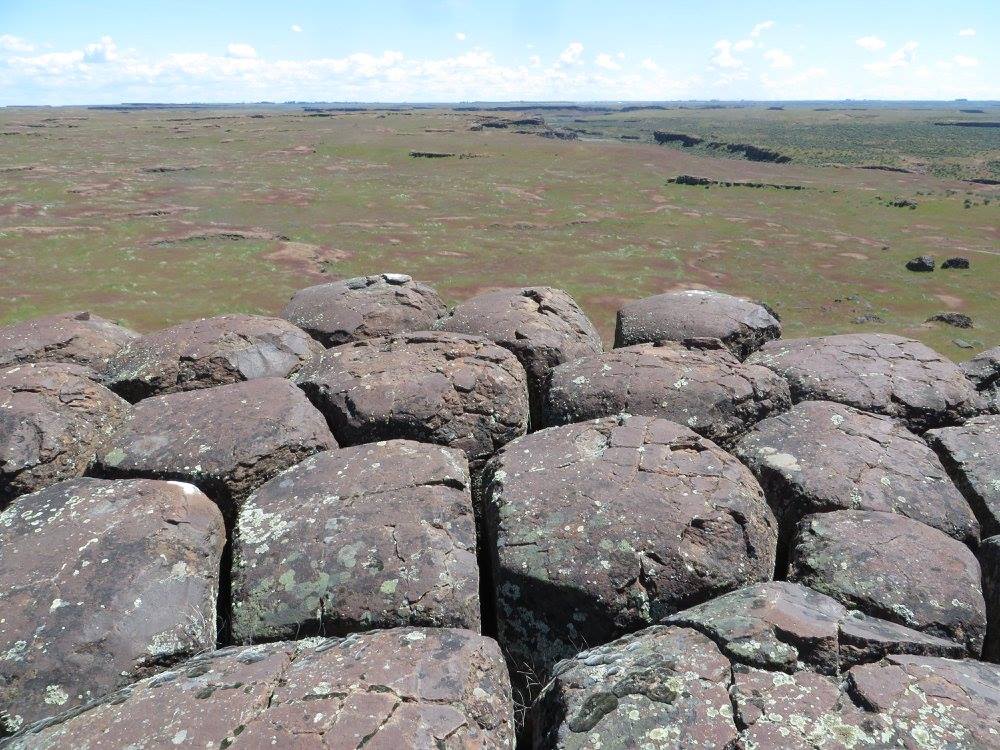
One delightful example of columnar basalt is at Svartifoss, the Black Waterfall, in southern Iceland. This is a magical little enclave. A distinctive feature is that the columns fracture and drop from the bottom up, forming an overhang with hundreds of small roofs. As the blocks break off and drop to the slope below, they assemble in a talus slope of hexagonal boulders. Hexagons are common in nature. Snowflakes, the cells of a honeycomb, pomegranate seeds, the many facets of an insect’s eye, and even carbon molecules are all hexagonal. Next time you are in a bathtub, take a good look at bubbles; while a single bubble is circular, the interior bubbles in a raft will morph to hexagons. This is a manifestation of the dense packing principle, which involves the most efficient way to fill a space with the least amount of material.
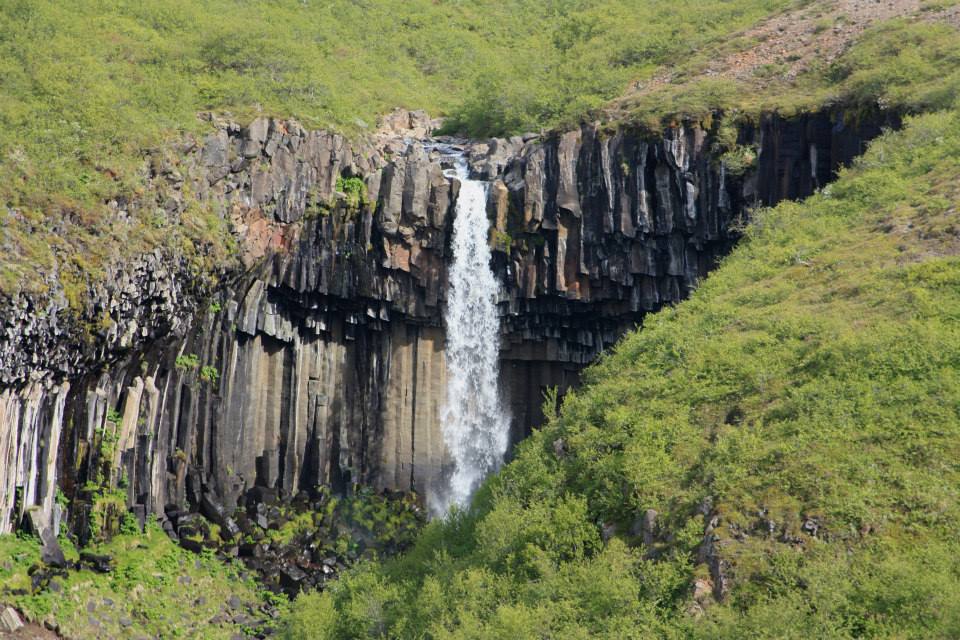
It would take many lifetimes to investigate the smorgasbord of basalt in Iceland. One good place to sample the variety of structure and texture is along the rugged coastline, where basalt cliffs meet the relentless pounding of the North Atlantic. It is possible to see how different layers of basalt take on different characteristics. The water exploits weaknesses in the rock, sculpting towers, caves, and arches. One of the best examples of columnar basalt in Iceland is the long cliff at Gerduberg, on the Snæfellsnes Peninsula. There is something deeply satisfying about standing at the base of basalt columns, staring up at the parallel cracks. There is something even more satisfying about climbing them!
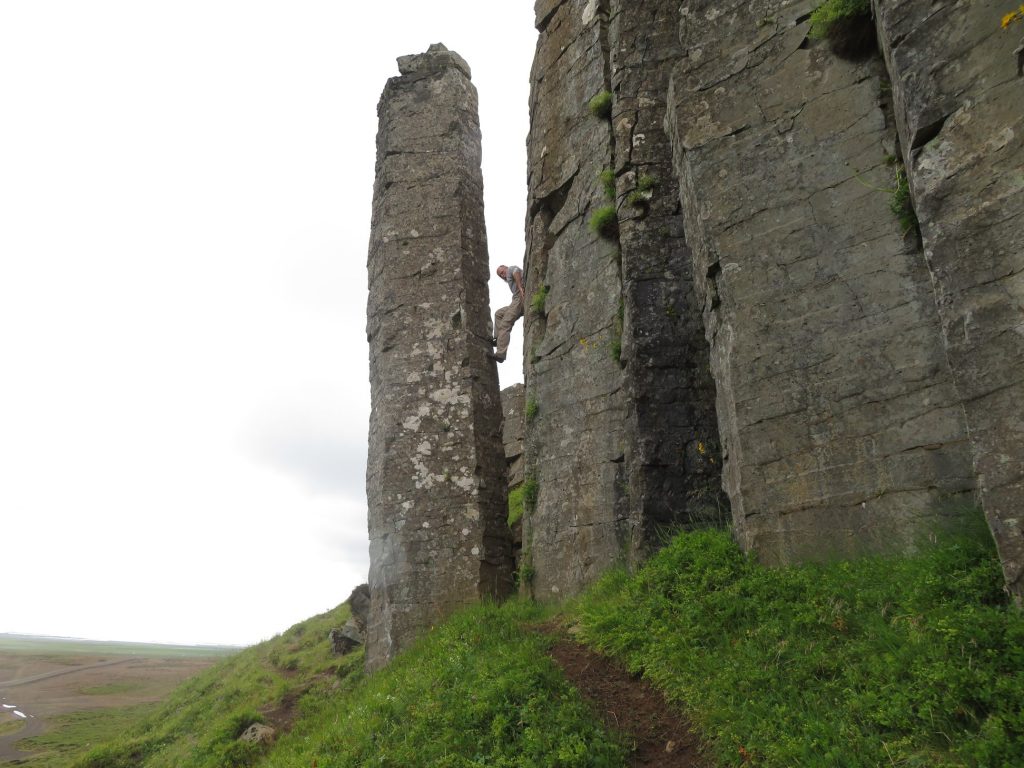
Basalt columns occur in volcanic landscapes all over the world. Three of the most famous formations are the Devil’s Postpile, in California; Devil’s Tower, in Wyoming; and the Devil’s Causeway, in Northern Ireland. I don’t know why the devil has such a fondness for columnar basalt. I find nothing sinister in these magnificent columns. Much of my home state of Washington is covered in basalt, due to the huge prehistoric effusion of lava known as the Columbia Basin flood basalts. A connoisseur of columnar basalt has much to choose from. Frenchmen Coulee is well-known for its tall basalt columns, many of them curiously rippled like crinkle-cut French Fries. The Tieton River valley offers miles of beautiful columns, tinged orange by lichen, rising above the beautiful swift-flowing Tieton River. But my favorite columnar basalt in Washington may be a crag at a place called the Drumheller Channels, just south of Moses Lake, in the desert interior.
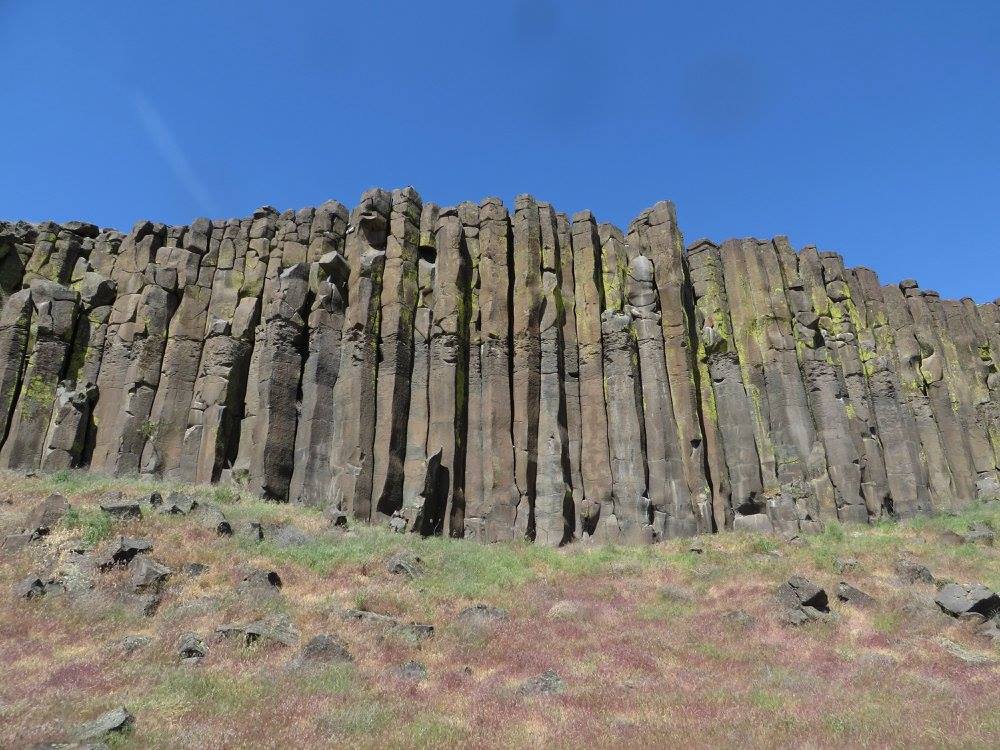
At Drumheller Channels, a long row of columns rises out of the grasslands. The top of the crag is a mosaic of basalt pillows separated by deep cracks. (Don’t drop your car keys!) It is a hike across a few miles of tick-infested grasslands to reach these cliffs, so it is very quiet and peaceful. From the top, the view over the channeled scablands feels oceanic. Approximately 15,000 years ago, unimaginably huge floods from glacial Lake Missoula scoured this country, carving deep coulees and countless potholes.
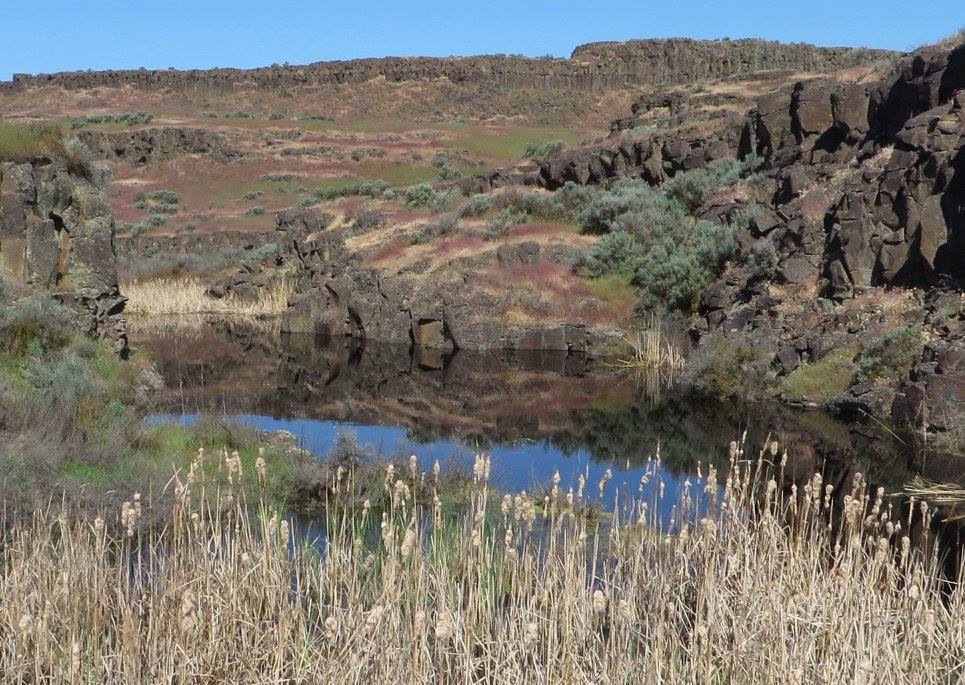
At the beginning of this SIBE, I mentioned that basalt has some interesting characteristics. I’m about to go into detail about one of those characteristics. It has to do with a chemical reaction that takes place between basalt and carbon dioxide. But first, let me say this: At my local plant nursery I can find basalt, ground to a fine powder and put in a bag, ready to add to my garden soil as an amendment. What is the benefit of that? Well, in theory, it adds some minerals that are important for root development and the uptake of nutrients and water. Many people know that volcanic soil, as it breaks down, is often quite fertile. In particular, basalt is high in both calcium and magnesium, which are important for plants. In practice, I consider the value of basalt dust as a garden amendment to be slight, although the people who put it in bags would beg to differ.
Which isn’t to say I’m not a fan of basalt dust. On a world-wide scale, and over the course of millennia, there is no denying the virtues of basalt-derived soil. It is often the substances that seem most humble and unremarkable that in fact hold properties that enable life and keep the earth in balance. One of the intriguing properties of basalt is this: As it weathers, it actually absorbs CO2 from the atmosphere, and transforms it to calcium carbonate in the form of calcite crystals. In effect, it changes CO2 from a gas to a solid. This property of basalt is gaining more attention in our time of runaway climate change.
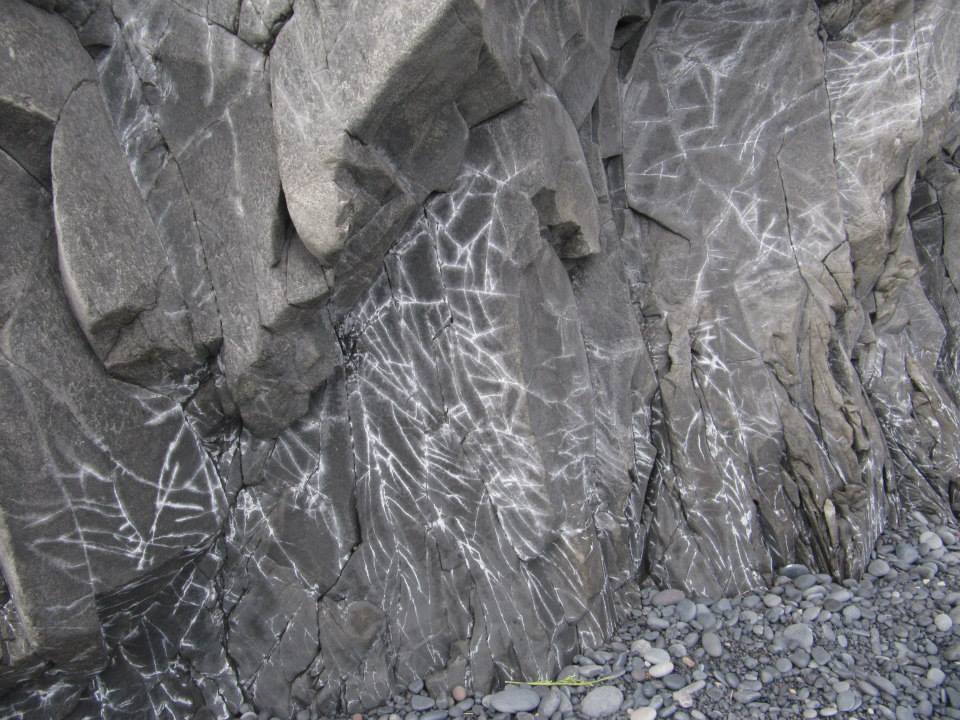
Enhanced Rock Weathering is gaining more attention as one proposed method of removing CO2 from the atmosphere and putting it back in soil. We all know what weathering refers to: the natural processes, both chemical and physical, that turn rocks into dirt. “Enhanced weathering” is a fancy term for speeding up the process. When proponents of ERW speak of weathering, the weathering they intend to “enhance” is primarily the breakdown of basalt. The way to enhance basalt it is to pulverize it. To make, essentially, a whole lot of basalt dust, and then get a whole lot of farmers to willingly spread it over a whole lot of fields. Maybe it could even be bagged and sold at your local nursery.
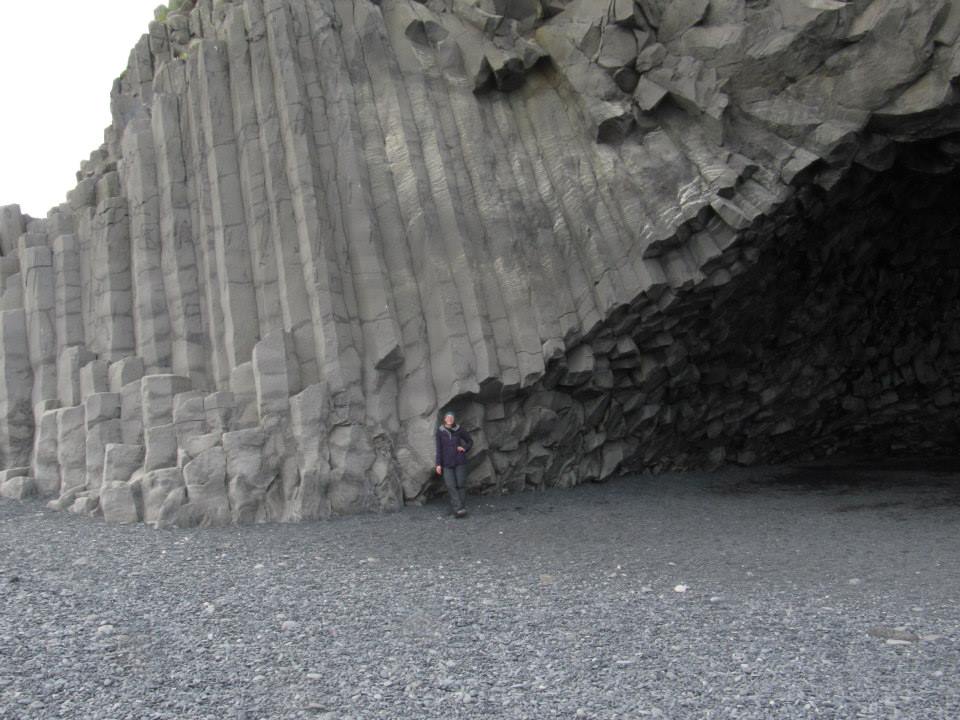
It almost sounds too simple to be true: You can grind basalt into powder, spread it on crops, and it not only benefits crops (well, maybe)—it also takes CO2 out of the air, as well. If the practice was wide-spread enough to bring this basalt dust downstream and into the ocean, it might also help estuaries by making the PH of the water slightly more alkaline. It sounds sort of like a crazy idea, to try to save the planet by smashing basalt. (I have vastly oversimplified the issue by putting it into these terms.) The reasoning is this: The process of CO2 sequestration through the weathering of basalt is already happening in nature, but it happens slowly and the effects are too small to address the degree of CO2 that is currently plaguing us. Why can’t we speed it up? Could it work? I don’t know, but it is being studied by serious people.
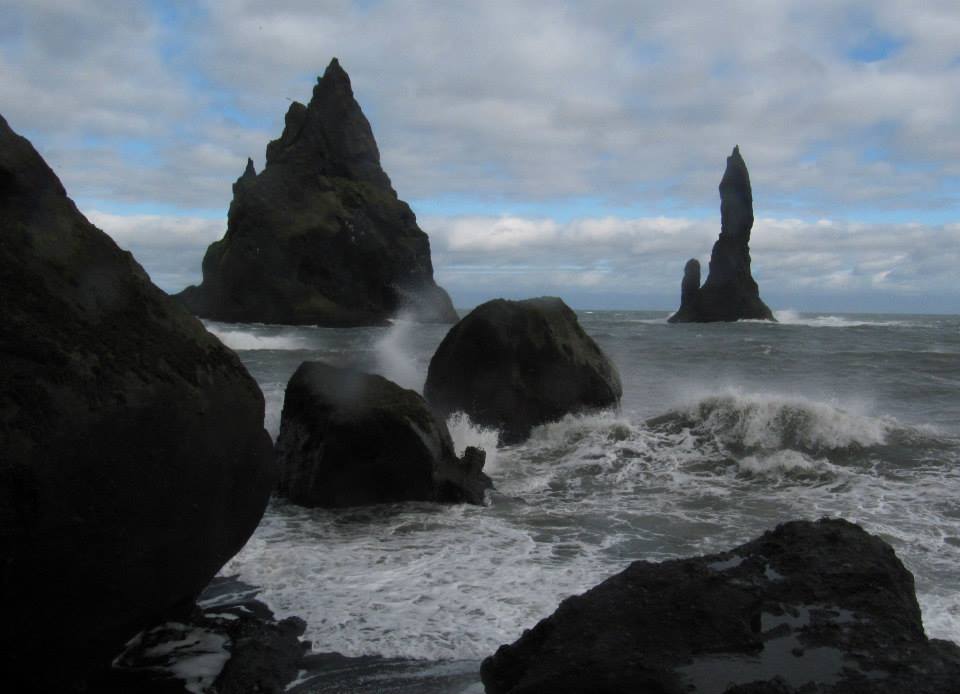
As it turns out, this stone that chronicles a time when almost all life on earth was wiped out due to a climate drastically altered by greenhouse gases, offers us some possible avenues to try to stop those same disastrous climatic changes from happening again. There is a wonderful geological irony to it. In the 4.5-billion year history of the earth, there have been a few catastrophes that have set back life in a major way. Most of these extinction events, as they are called, have been associated with the same large-scale vulcanism that gives us LIPs and most of our basalt. In fact, the worst extinction event happened at the end of the Permian era, about 250 million years ago, and was due to the extravagant vulcanism that created the Siberian Traps. How interesting that the type of rock that is most associated with extinction-causing climate disasters is also the type of rock that, over time, sets things right again.
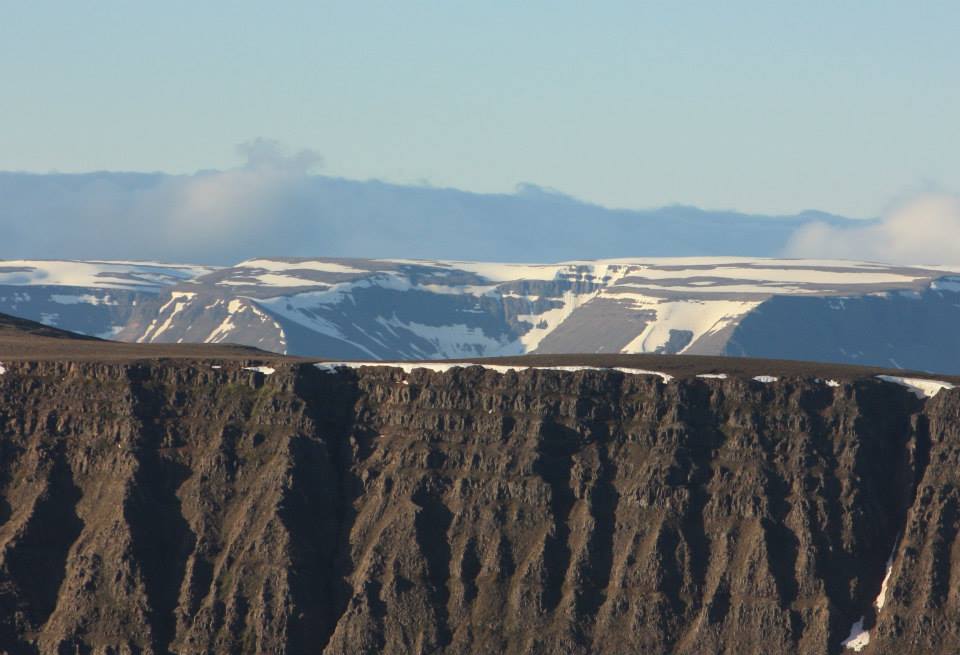
In the natural cycle of vulcanism, the weathering of the basalt produced in atmosphere-altering eruptions is a long-term mechanism through which the greenhouse gases from those very eruptions are reduced. On a geologic time scale, basalt-derived soil does indeed absorb atmospheric CO2 and transform it to calcium carbonate. In due course, the alkaline calcium carbonate finds its way to the sea and gradually brings acidic oceans back into balance. But it takes a long damn time. How long? Well, far, far longer than Homo sapiens has (so far) walked the earth. Geologists estimate that it took nine or ten million years for life to bounce back after the Permian extinction, when no one was around to smash up the basalt.
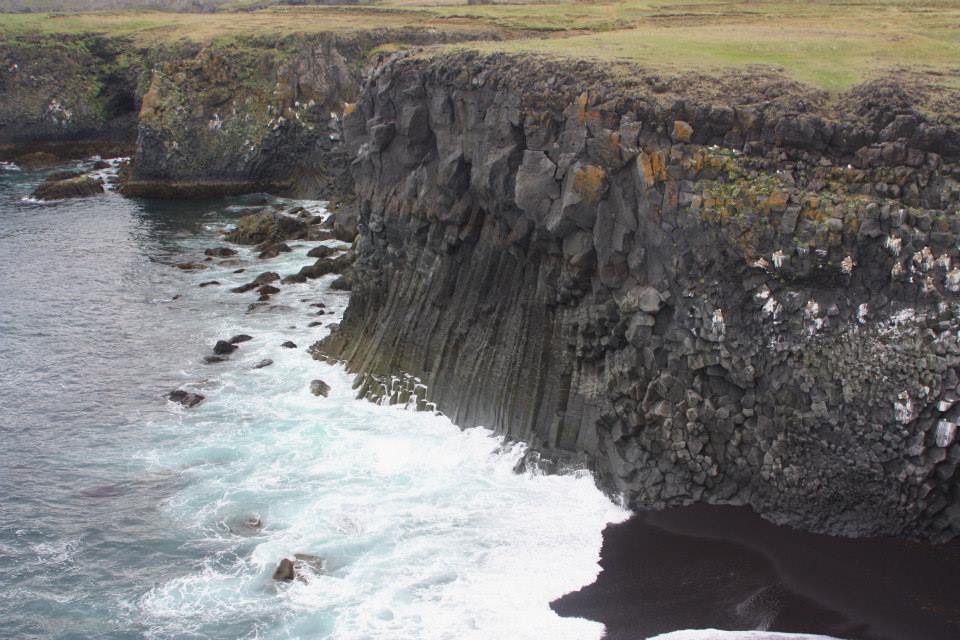
At the time of the Permian extinction event, the concentration of CO2 in the atmosphere was probably at least twice what it is now. This was sufficient to kill 85 to 90% of terrestrial species, and 95% of marine species. How high is the concentration of CO2 in today’s atmosphere? In 2021, it reached 421 parts per million (PPM). Although not as high as it was during the Permian Extinction, this is definitively higher than it has been at any point in the past 800,000 years, probably higher than it has been in the past 3 million years, and possibly higher than it has been in the past 23 million years.
On the climb up to that high concentration at the end of the Permian era, it was a relatively slow climb until certain tipping points were reached; then it accelerated. If we are educated about what is happening in our world currently, we are familiar with some of these tipping points: The release of methane from the sea floor. The thawing of permafrost. The loss of Arctic ice. Unless we change direction decisively, we are likely headed into an extinction event as severe as any the earth has yet experienced. Will it be “game over” for the earth itself? Of course not. Basalt, for instance, will endure. Water will endure—although most of the life in it may not. But it will be game over for the vast majority of plants and animals. How will humans fare? Who knows? But considering that we depend on other living creatures for our survival, the outlook is poor.
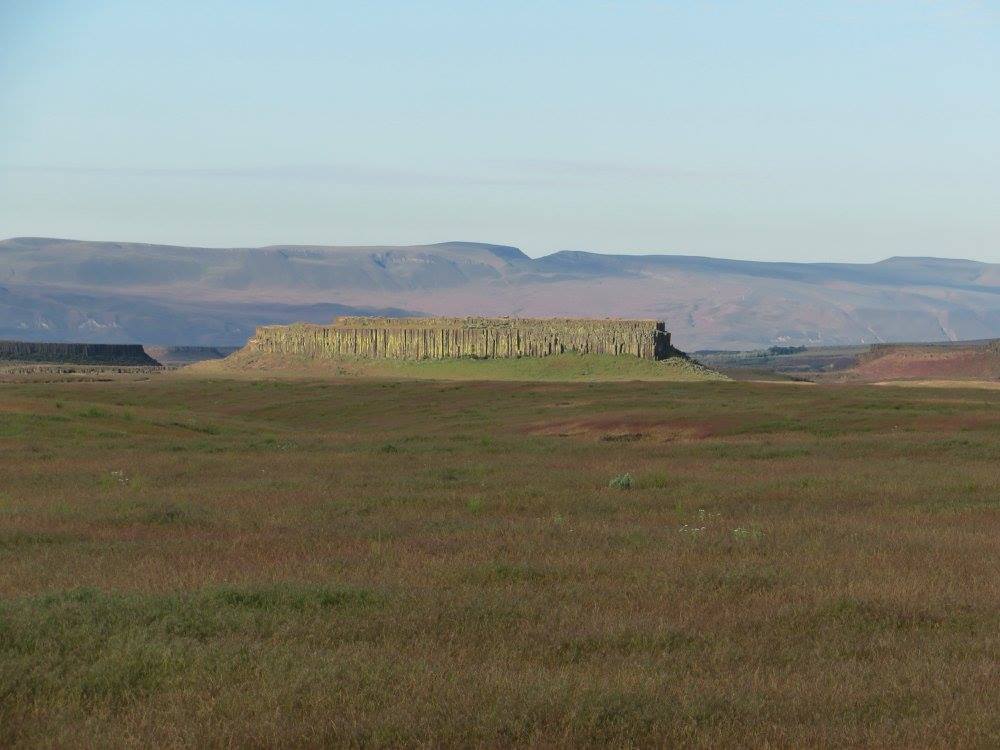
Of course an extinction “event” doesn’t happen all at once. In fact, it’s not a single event. It doesn’t happen on a Tuesday at 3:40 PM. In fact, it can’t even be pinned to a specific year or even decade. The event we are in now is not unfolding quickly enough to compete with news headlines about Covid-19 or Afghanistan—but it is of greater consequence than anything else that makes the news. Nothing else even comes close. It’s hard to predict how long it will take, although on a geologic time scale it is happening drastically fast—far more quickly than any previous extinction event caused by volcanic activity.
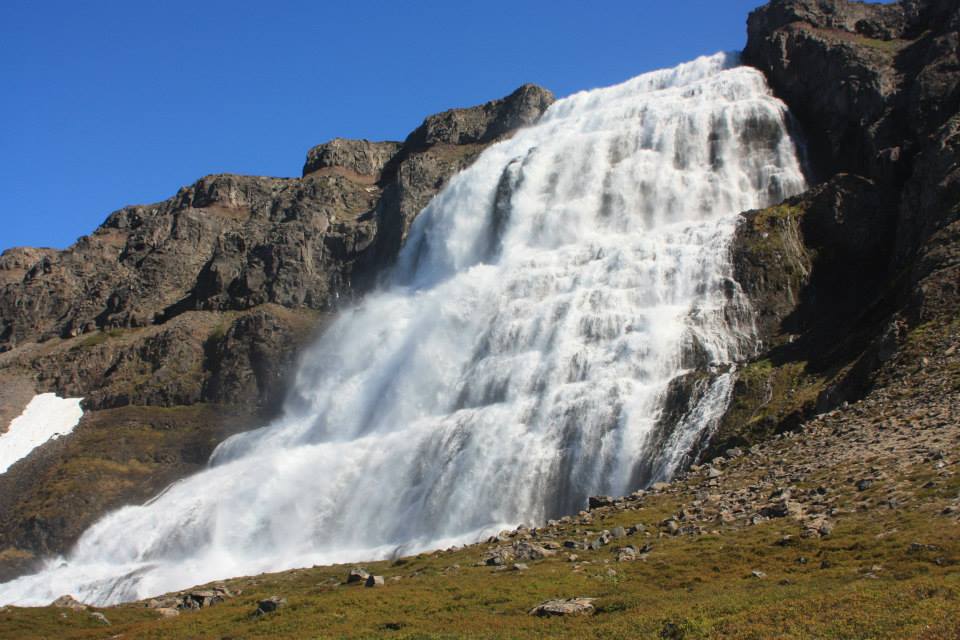
How much “enhanced weathering” of basalt would it take to make a difference? A helluva lot. How much would it benefit crops or estuaries? Not clear. Is this a silver bullet? No. There are no simple answers to the problem of climate change. The problem is so large that our efforts to combat it must be correspondingly large. It is increasingly clear that modern human societies are not changing quickly enough to slow down greenhouse gas emissions in the next couple of decades. We must find ways not only to decrease what we put into the air, but also to remove what we have already put into it. I don’t know what the best solutions will be, but it seems to me that we need to look to natural processes for insight. In that regard, basalt has something to say to us—whether we pulverize it or not. If we listen carefully, the soil itself may tell us what to do. The water, too, may tell us.
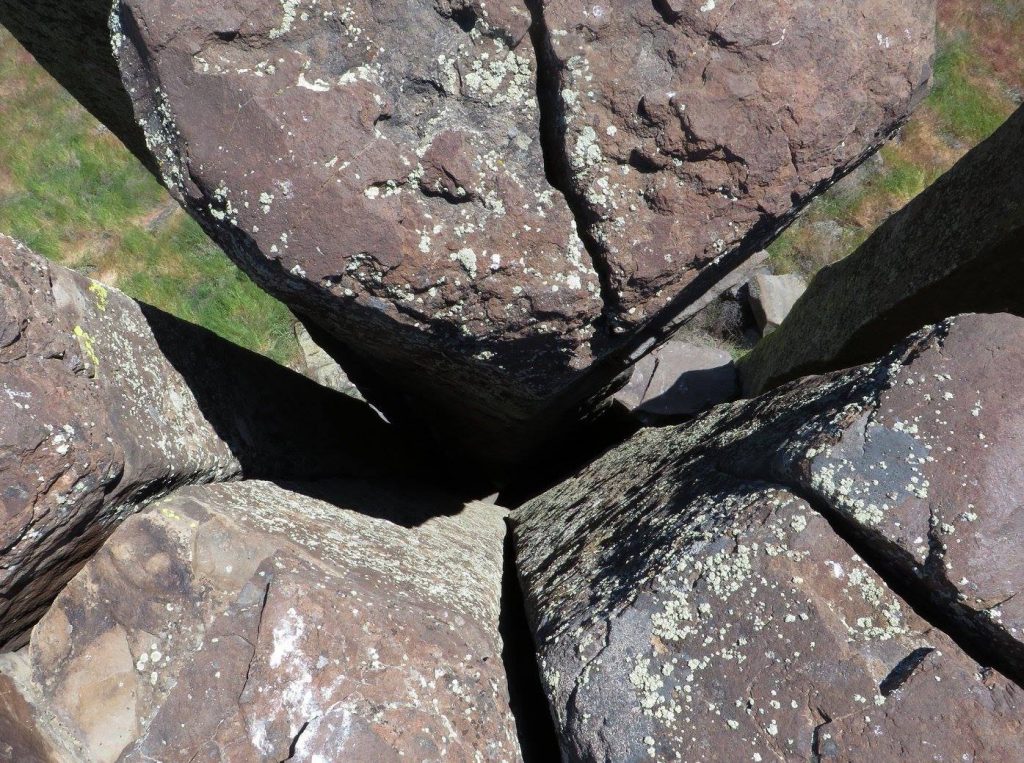
I do not know if basalt can be a key to unlock at least a partial solution to climate change. I do know that basalt is, to me, a synecdoche of the earth herself. It is both the question and the answer. It is Brahma, Vishnu, and Shiva, the beginning, the middle, and the end. And it looks so good in columns.
***
I don’t have a public comments option, but I welcome your responses. Feel free to leave a comment at markrozema62@gmail.com